
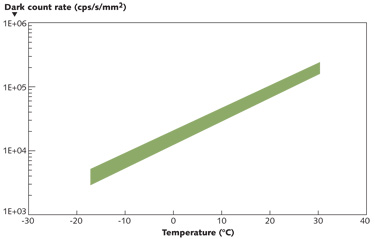
The dark current of the AlGaAsSb APDs reported here is also much lower than reports of low noise AlInAsSb SAM APDs (6.1 mAcm -2) using an Al 0.4In 0.6As 0.3Sb 0.7 absorber 5. At a gain of 10, the bulk dark current of the AlGaAsSb APDs is just 13 nA, which is comparable to mature commercial InGaAs APDs by Hamamatsu (20 nA) 9, Excelitas (45 nA) 10 and Laser Components (25 nA) 11. The 230 μm diameter APD allows for a circular optical window of diameter 200 μm, the bulk dark current can be compared to mature commercial InGaAs APDs also with an optical window of 200 μm. We find that the total dark current is dominated by surface current at voltages less than 45 V and bulk current at > 45V. 8 The unmultiplied and multiplied dark currents of the 230 μm diameter APD were found to be 18 nA and 1.3 nA respectively over a voltage range of 36 to 44V corresponding to gain values of 3.5 to 20. I surface and I bulk were calculated by fitting the two components across a range of voltages using a linear regression between dark current and gain using equation 2. Typically, the unmultiplied component of the leakage is due to surface effects and the multiplied is due to bulk related leakage current usually stemming from the low band gap material. To investigate the source of the leakage current, the unmultiplied (I surface) and multiplied (I bulk) components of the dark current were calculated. The 230 μm diameter APDs had an average dark current of 31 nA at a gain of 10, equivalent to a current density of 74.6 μAcm -2. Gain is calculated by dividing the responsivity by the unity gain responsivity. The device used is a 230 μm diameter circular mesa. Room temperature dark current (black, left axis), photocurrent (red, left axis) and avalanche gain (blue, right axis) versus reverse voltage for the InGaAs/AlGaAsSb SAM APD. The experimental setup is described elsewhere in further detail 7. Responsivity and gain results were also corroborated against the theoretical value for responsivity which was calculated using the absorption coefficient of InGaAs 6 and accounting for reflections from the optical window.Įxcess noise measurements as a function of gain (F(M)) were carried out by phase-sensitive detection and a modulated 940 nm LED. Unity gain and responsivity of the APDs were validated against a reference photodiode with the same absorption layer thickness.
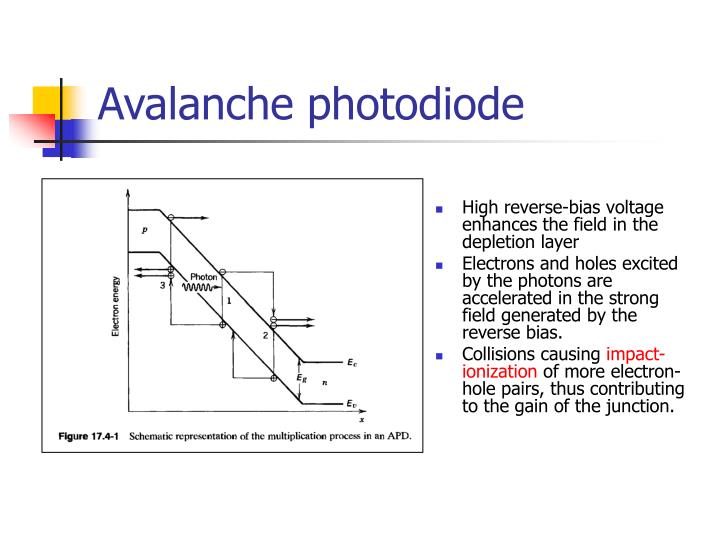
The gain was verified to be the same for both 940 nm and 1550 nm, which is expected as both 940 nm and 1550 nm are solely absorbed in the InGaAs absorber, providing pure carrier injection into the AlGaAsSb multiplication region. Gain measurements were performed using phase-sensitive techniques using a lock-in amplifier and chopped He-Ne laser at 1550 nm and modulated LED at 940 nm. The optically active part of the APD is an 80 μm-diameter window in the center of the die. (a) A schematic diagram of the layer structure of the APD (b) An image showing a single APD die bonded onto a ceramic header. The prospects for the next generation of extremely low noise APDs for 1550 nm light detection are discussed. We discuss the impact of the excess noise, dark current and responsivity on the APDs sensitivity and, project a noise-equivalent power (NEP) below 80 fW/Hz 0.5 from a 230 μm diameter APD and commercial transimpedance amplifier (TIA).

Furthermore, the APD’s dark current density was measured to be 74.6 μA/cm 2 at a gain of 10 which is competitive with commercial devices. In this paper, we report on the first low noise InGaAs/AlGaAsSb separate absorption, grading and multiplication APDs operating at 1550 nm with extremely low excess noise factor of 1.93 at a gain of 10 and 2.94 at a gain of 20. Recently, we have demonstrated extremely low noise from an Al(Ga)AsSb PIN diode with highly dissimilar impact ionization coefficients due to electron dominated impact ionization. The underlying issue is that the impact ionization coefficient of InAlAs and InP used as the avalanche region in current commercial APDs are very similar at high gain, leading to poor excess noise performance. It is well-known that an avalanche photodiode (APD) can be used to improve the signal to noise ratio over a PIN detector, however, APDs operating at the eye-safe wavelengths around 1550 nm are limited in sensitivity by high excess noise. The optical detector used in pulsed LIDAR, range finding and optical time domain reflectometry systems is typically the limiting factor in the system’s sensitivity.
